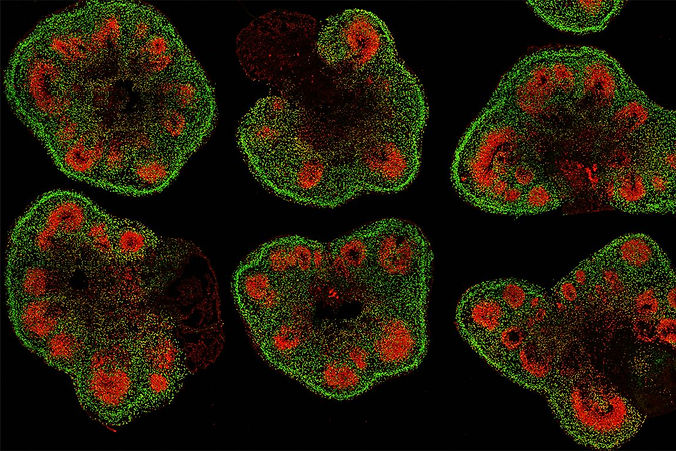
An Introduction to the Ethics of
Brain Organoids
"If you look at the anatomy, the structure, the function, there's nothing in the universe that's more beautiful, that's more complex, than the human brain." - Keith Black
Human brain organoids are a subject of recent ethical discussion because they exhibit electrical activity reminiscent of human brain activity. This property raises the question—could these tiny clusters of cells be conscious? Some argue that current brain organoids do not have the capacity for consciousness, while others insist that we can not rule out that brain organoids are conscious. Just because there is ion movement in the cells does not mean that the tissue possesses the same complexity of neural networks needed for consciousness. However, science must also work within the bounds of what is morally acceptable to society. Explore this page to learn more!
The Basics of Brain Organoids
Human brain organoids are tiny three-dimensional cell cultures that enable scientists to model and understand the brain in vitro (Kelava & Lancaster, 2016). The brain cells that self-organize into these tiny, complex clumps typically derive from pluripotent cell sources—“blank slate” cell sources capable of becoming many different kinds of mature brain cells (Lavazza, 2021). The two most common pluripotent cell sources are embryonic stem cells derived from early-stage embryos and reprogrammed adult cells called induced pluripotent stem cells. By adding specific signaling factors, such as transforming growth factor-beta, sonic hedgehog, or retinoic acid, researchers can produce many different types of brain organoids from these pluripotent cells, modeling different regions of the human brain. Some regions that have successfully been modeled with organoids include the hippocampus, midbrain, pituitary, hypothalamus, and cerebellum (Jo et al., 2016; Sakaguchi et al., 2015; Ozone et al., 2016; Qian et al., 2016; Muguruma et al., 2015).

Brain Organoids
So how do researchers create brain organoid models? Nervous tissue derives from the ectoderm germ layer, so many researchers start there (Lavazza, 2021). They might add ectodermal cells to nutrient-rich matrigel droplets in a rotating bioreactor. Wait ten days, and the organoid develops neurons. Wait thirty days, and the organoid self-assembles into a layered structure that resembles a developing human brain. As Dr. Cleber Trujillo of the Sanford Consortium for Regenerative Medicine describes, “We don’t make [brain organoids] by putting cells together. We just give cells the right conditions to grow, and they organize themselves.” He makes the process sound simple, but scientists have optimized organoid protocol for years. Even today, they continue to refine their processes to generate diverse brain organoid models with unique properties.
Brain organoids only grow to roughly 4-5 mm across because they typically do not have blood vessels.
Brain organoids exhibit several exciting features, including the capacity to receive sensory inputs and send functional outputs (Gabriel et al., 2021; Giandomenico et al., 2019). At a cellular level, organoids are comparable to an in vivo developing brain (Kelava & Lancaster, 2016). Moreover, evidence supports that brain organoids can display synchronized electrical activity and demonstrate a form of learning (Kagan et al., 2022; Sakaguchi et al., 2019). One research group even demonstrated that the brain waves observed in cerebral organoids resemble the brain waves observed in premature infants (Trujillo et al., 2019). These findings excite researchers because they recapitulate (to an extent) the characteristics of a human brain. This makes brain organoids a revolutionary advancement for neuroscience research because organoid models can overcome the limitations of small animals and cadavers, allowing researchers to delve deeper into neurophysiology and brain conditions than ever before. For example, researchers use brain organoids to study neurodevelopment and neurodevelopmental disorders, understand the disease mechanisms of neurodegenerative, neurological, and psychiatric conditions, probe evolutionary biology questions, and screen drugs for treatment efficacy (Kelava & Lancaster, 2016).
​
Despite their efficacy as an experimental model, brain organoids are not as sophisticated as the human brain. Though the science is advancing rapidly, many researchers agree that current organoids lack the cell type diversity, sensory inputs, and complex network structure necessary to replicate a human-like subject experience (Hyun et al., 2020). Developed brain organoids are also incredibly small—only growing to approximately four to five millimeters in diameter because they typically lack the blood vessel networks necessary to supply cells at the core of the organoid with nutrients, oxygen, and waste removal (Lavazza, 2021). Such brain organoid limitations are active areas of research. Scientists are constantly trying to make better brain organoid models. Ultimately, this work leads to exciting discoveries with the potential to improve human health and raises ethical issues surrounding the capacity of future brain organoids to develop consciousness.
Learn how University of San Diego researchers create brain organoids from pluripotent stem cells.
Listen to Dr. Madeline Lancaster, a leader in brain organoid research, explain the advantages and limitations of these model "mini brains."
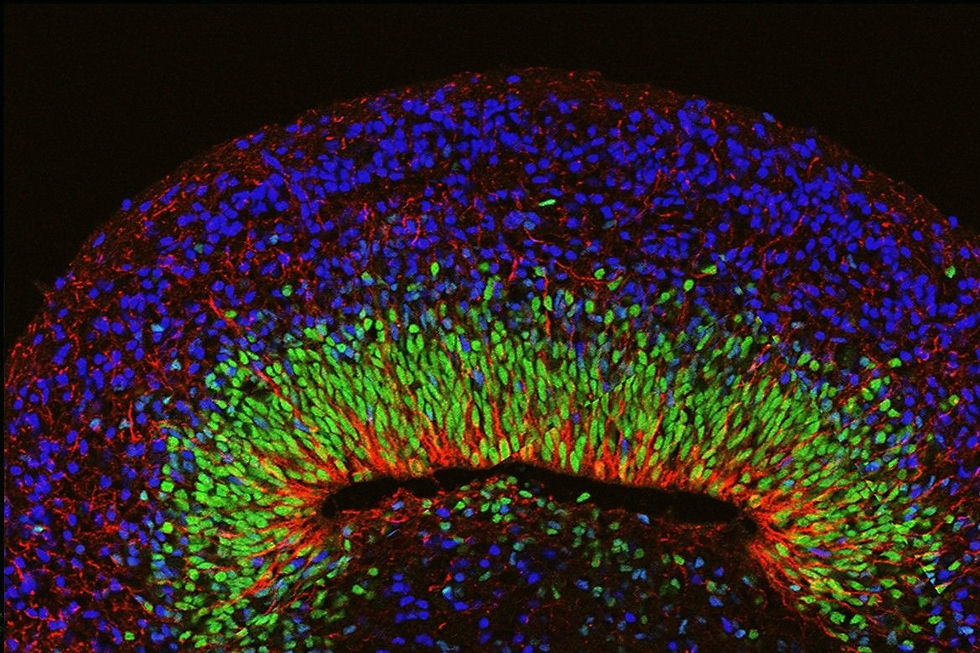
Research Applications for Brain Organoids
Understanding Brain Development & Evolution
Because the adult human brain consists of billions of neurons and glial cells arranged in a complex, interconnected pattern, scientists are eager to understand how brain circuitry patterns begin during fetal and embryonic development. They study early neurogenesis in 3D brain organoid culture by monitoring the cells as they self-organization into structures that resemble human development. For example, the ventricular zone, subventricular zone, and cortical plate can all be identified in later stages of human brain and organoid development. Beyond recapitulating typical brain development, researchers model neurodevelopmental disorders, such as autism spectrum disorder, to better understand their mechanisms. One group studying brain organoids created from patients with autism spectrum found that the patient-derived organoids exhibited increased expression of the FOXG1 gene, which increased GABAergic neurons.
Source: Kelava & Lancaster, M. A. (2016) and Mariani et al. (2015)
Brain-Related Disease Modeling
Brain organoid modeling can overcome the limitations of modeling diseases with small animals, like mice and rats. Such research has been applied to understanding neurodevelopmental disorders, neurodegenerative disorders, neurological disorders, and mental illness. For example, researchers studying a mutation in a gene called CDK5RAP2 found that mice did not exhibit microcephaly—a smaller brain than expected—like humans do with this genetic variant. When they sampled fibroblast cells from an individual with microcephaly and reprogrammed them into neural tissue, they observed that the resulting organoids were smaller than brain organoids derived from individuals without microcephaly. The scientists identified why the organoids were different. The areas of replicating cells in the organoids without microcephaly were aligned for maximal growth, whereas the alignment in the organoid from a person with microcephaly was not optimized.
Source: Kelava, I., & Lancaster, M. A. (2016).
Drug Development & Screening
Brain organoids present exciting opportunities for drug screening and development. Namely, potential drug compounds for brain conditions can be tested on brain organoids to identify efficacious compounds before animal or human testing. Furthermore, patient-specific brain organoids can be produced for personalized medicine—beyond general, high-throughput drug screenings to find potential treatments for various conditions. This process can work by reprogramming fibroblast cells from a patient’s urine sample to induced pluripotent stem cells. Consequently, the brain organoid cells will contain the patient’s DNA, and individualized therapy can be determined. With the development of scalable bioreactors, like SpinΩ systems, for paralleled mass production of organoids, these drug screening applications are becoming more and more feasible for pharmaceutical research and clinical applications.
Source: Kelava, I., & Lancaster, M. A. (2016).
Should brain organoids be used for research if they have a capacity for consciousness?
If future brain organoids develop sentience—the ability to feel pain and pleasure—would this change how scientists conduct research? Would it be ethical to experiment on organoids with drugs or potentially painful stimuli? Would it be okay to leave organoids in a lab dish to die? Suppose that beyond being able to feel pain and pleasure, future organoids exhibited signs of the subjective phenomenon we call consciousness. What types of brain organoid research would be appropriate then? These types of questions keep ethicists up at night—and not all ethicists agree. Some ethicists argue that scientists should treat any brain organoid with the essential structures for consciousness as conscious. This conservative argument follows the precautionary principle (Birch & Browning, 2021). Others express far less concern. To understand the ethical dilemma of what organoid consciousness might mean for research, let’s consider both sides of the issue—should brain organoids be used for research if they develop a capacity for consciousness?
Yes, brain organoids should still be used for research.
It must be ethically permissible to experiment with brain organoids with the capacity for consciousness because current research uses conscious subjects. Research labs test therapies on small rodents. While some might argue that the consciousness of rodents differs from human consciousness, research studies experiment with non-human primates and humans too. Thus, unless all in vivo animal and human research is unethical because animals and humans possess the capacity for consciousness, then why would organoid experiments be unethical? Why would brain organoids with the potential for a level of consciousness arguably lower than human consciousness receive better treatment than humans​? We justify non-human and human research when the potential benefit to society (i.e., understanding diseases or how to treat them) outweighs the potential harm incurred by the study participant. Brain organoid research demonstrates immense value for advancing our understanding of neurophysiology and brain-related conditions, meeting this standard.
Viewpoint presented by Hank Greely, a Professor of Law at Stanford University
No, brain organoids should not be used for research.
It should be assumed that it is not ethically permissible to experiment with brain organoids with the capacity for consciousness because we cannot rule out that such entities have high moral status. Without the knowledge of the subjective states of cerebral organoids, we cannot ethically perform experiments that may lead to sensations of pain or discomfort. For example, a researcher would never dissect a human brain without ensuring the patient had died because our society assigns moral value to living beings. Thus, we must act conservatively and proceed with caution.
Viewpoint summarized by Dr. Karola Kreitmair, a Medical Historian and Ethicist at the University of Wisconsin-Madison
A lecture on cerebral organoid consciousness by Dr. Christof Koch, a neurophysiologist with the Allen Institute for Brain Science.
A brain organoid ethics panel discussion, including input from neuroscience and philosophy experts Christof Koch, Patricia Churchland, Evan Thompson, and Alysson Muotri.
Brain Organoid Researcher Perspectives
So where do brain organoid researchers stand on the ethics of their work? Do the same questions that ethicists consider keep them up at night too? An in-depth interview study by Dr. Andrea Lavazza and Dr. Alice Chinaia suggests that scientists recognize the importance of discussing organoid ethics (Lavazza & Chinaia, 2023). Lavazza and Chinaia’s systematic research project evaluated the perspectives of biologists, bioengineers, neurologists, and neurosurgeons with at least two years of experience working with cerebral brain organoids. Their results revealed several shared perspectives. First, most brain organoid researchers remain skeptical that brain organoids can develop a capacity for consciousness. Many scientists who participated in the study agreed that brain organoids lack the size, complexity, and environmental interactions necessary for developing such a state. They also questioned the validity of existing definitions of pain and consciousness, explaining that they are too vague to apply to their work confidently. Moreover, regarding the moral status of brain organoids, researchers tended to believe that even if brain organoids developed some form of consciousness sometime in the future, their sentience would be comparable to flies. Even then, the most cautious researchers suggested that brain organoid research could still continue.
​
Second, brain organoid researchers demonstrated a desire to engage in ethical discussions surrounding their work, even if they do not worry as much about the issues of organoid consciousness and moral status as the ethicists. Specifically, researchers emphasized the need for better communication between researchers and the public about the science of brain organoids. They worry that the media can misconstrue brain organoid science, potentially exacerbating public concern over the moral implications of so-called “mini brains.” Namely, the portrayal of mini human brains growing in laboratories in media coverage often makes these clumps of cells seem “more alive” and more human-like than cells growing in a simple Petri dish. For this reason, some researchers even reject the terms “mini-brains” or “brains in a dish” to describe these models. They see brain organoids as just another type of cell culture. Thus, they are eager to engage in dialogue to ensure the public is more informed about brain organoid research progress—that brain organoids do not yet exhibit the same complexities as a human brain.
Brain in a Vat
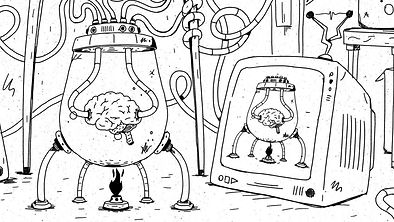
A Thought Experiment
Is everything you believe to be true simply an illusion? Could your existence be a brain in a vat, fed information that simulates your experiences? And if you were a brain in a vat, would it be possible to know? Meet the "brain in vat" thought experiment, a debate that has puzzled philosophers since Descartes' famous "I think, therefore I am." At its core, the "brain in a vat" questions our knowledge of our lived reality (Bernicker, 2016). We're prompted to consider our definitions of truth, mind, and meaning. Sound like a science fiction movie? That's because the basis of the "brain in a vat" appears in movies like The Truman Show, and keeping a brain alive in a vat sounds like the work of a stereotypical mad scientist. It leaves us grappling with the notion that we cannot truly know what we know with certainty, and we cannot rule out the possibility that our existence is a simulation. Whether the "brain in a vat" thought experiment sends your mind in circles or sparks your curiosity, it provides one way of thinking about the potential for brain organoid consciousness like a philosopher (Lavazza, 2020). We can entertain the idea that a brain organoid with the capacity for consciousness might be comparable to a state of human consciousness.
Learn How Researchers Use Organoids to Understand the Brain
"By allowing us to recreate circuits of the human brain, we will gain new insights into human biology. This in itself will open a new era in the treatment of brain disorders." - Dr. Sergiu P. Pasca, Stanford University
The Future of Brain Organoid Research
Brain organoid science is progressing rapidly. Scientists continue to learn more about reprogramming stem cells and providing organoids with the signals they need to develop similarly to a human brain. They are experimenting with making blood vessels grow in organoids and using microfluidic devices to control signaling molecule delivery (Kelava & Lancaster, 2016). Improved vascularization promises to overcome current brain organoid size constraints, enabling researchers to produce bigger organoids, and, more importantly, allow researchers to study the blood-brain barrier. With these and other advancements, Dr. Madeline Lancaster—a leader in the field of brain organoids—predicts that organoids may fully mimic tissue development in vitro, along with adult brain disorders and brain conditions associated with aging within two decades.
​
There are many other unique and exciting areas of progress. Examples include...
-
Combining brain organoids with robotics and artificial intelligence
-
Integrating neuron networks and multi-electrode arrays and teaching them to play games,
-
Sending brain organoids to space to understand how neural development
​
As long as researchers and ethicists continue to consider the ethical and societal implications of brain organoids, these models demonstrate immense potential for improving our understanding and treatment of brain-related conditions through research, drug screening, personalized medicine, and beyond.
References
-
Bernicker, B. (2016, January 28). Brain in a Vat. Thought Experiments. https://sites.psu.edu/bernickerpassionblog/2016/01/28/brain-in-a-vat/
-
Birch, J., & Browning, H. (2021). Neural Organoids and the Precautionary Principle. The American Journal of Bioethics, 21(1), 56–58. https://doi.org/10.1080/15265161.2020.1845858
-
Gabriel, E., Albanna, W., Pasquini, G., Ramani, A., Josipovic, N., Mariappan, A., Schinzel, F., Karch, C. M., Bao, G., Gottardo, M., Suren, A. A., Hescheler, J., Nagel-Wolfrum, K., Persico, V., Rizzoli, S. O., Altmüller, J., Riparbelli, M. G., Callaini, G., Goureau, O., Papantonis, A., … Gopalakrishnan, J. (2021). Human brain organoids assemble functionally integrated bilateral optic vesicles. Cell Stem Cell, 28(10), 1740–1757.e8. https://doi.org/10.1016/j.stem.2021.07.010
-
Giandomenico, S. L., Mierau, S. B., Gibbons, G. M., Wenger, L. M. D., Masullo, L., Sit, T., Sutcliffe, M., Boulanger, J., Tripodi, M., Derivery, E., Paulsen, O., Lakatos, A., & Lancaster, M. A. (2019). Cerebral organoids at the air-liquid interface generate diverse nerve tracts with functional output. Nature Neuroscience, 22(4), 669–679. https://doi.org/10.1038/s41593-019-0350-2
-
Greely, H. T. H., & Kreitmair, K. V. (2021). Should Cerebral Organoids be Used for Research if they Have the Capacity for Consciousness?. The International Journal of Healthcare Ethics Committees, 30(4), 575–584. https://doi.org/10.1017/S0963180121000050
-
Hyun, I., Scharf-Deering, J. C., & Lunshof, J. E. (2020). Ethical issues related to brain organoid research. Brain Research, 1732, 146653–146653. https://doi.org/10.1016/j.brainres.2020.146653
-
Jo, J., Xiao, Y., Sun, A. X., Cukuroglu, E., Tran, H.-D., Göke, J., Tan, Z. Y., Saw, T. Y., Tan, C.-P., Lokman, H., Lee, Y., Kim, D., Ko, H. S., Kim, S.-O., Park, J. H., Cho, N.-J., Hyde, T. M., Kleinman, J. E., Shin, J. H., … Ng, H.-H. (2016). Midbrain-like Organoids from Human Pluripotent Stem Cells Contain Functional Dopaminergic and Neuromelanin-Producing Neurons. Cell Stem Cell, 19(2), 248–257. https://doi.org/10.1016/j.stem.2016.07.005
-
Kagan, B. J., Kitchen, A. C., Tran, N. T., Habibollahi, F., Khajehnejad, M., Parker, B. J., Bhat, A., Rollo, B., Razi, A., & Friston, K. J. (2022). In vitro neurons learn and exhibit sentience when embodied in a simulated game-world. Neuron, 110(23), 3952–3969.e8. https://doi.org/10.1016/j.neuron.2022.09.001
-
Kelava, I., & Lancaster, M. A. (2016). Dishing out mini-brains: Current progress and future prospects in brain organoid research. Developmental Biology, 420(2), 199–209. https://doi.org/10.1016/j.ydbio.2016.06.037
-
Lavazza A. (2020). Human cerebral organoids and consciousness: A double-edged sword. Monash Bioethics Review, 38(2), 105–128. https://doi.org/10.1007/s40592-020-00116-y
-
Lavazza, A., & Pizzetti, F. G. (2020). Human cerebral organoids as a new legal and ethical challenge. Journal of Law and the Biosciences, 7(1), J1–lsaa005. https://doi.org/10.1093/jlb/lsaa005
-
Lavazza, A. (2021). “Consciousnessoids”: Clues and insights from human cerebral organoids for the study of consciousness. Neuroscience of Consciousness, 2021(2), niab029–niab029. https://doi.org/10.1093/nc/niab029
-
Lavazza, A., & Chinaia, A. A. (2023). Human cerebral organoids: The ethical stance of scientists. Stem Cell Research & Therapy, 14(1), 59. https://doi.org/10.1186/s13287-023-03291-x
-
Mariani, J., Coppola, G., Zhang, P., Abyzov, A., Provini, L., Tomasini, L., Amenduni, M., Szekely, A., Palejev, D., Wilson, M., Gerstein, M., Grigorenko, E. L., Chawarska, K., Pelphrey, K. A., Howe, J. R., & Vaccarino, F. M. (2015). FOXG1-Dependent Dysregulation of GABA/Glutamate Neuron Differentiation in Autism Spectrum Disorders. Cell, 162(2), 375–390. https://doi.org/10.1016/j.cell.2015.06.034
-
Muguruma, K., Nishiyama, A., Kawakami, H., Hashimoto, K., & Sasai, Y. (2015). Self-Organization of Polarized Cerebellar Tissue in 3D Culture of Human Pluripotent Stem Cells. Cell Reports (Cambridge), 10(4), 537–550. https://doi.org/10.1016/j.celrep.2014.12.051
-
Ozone, C., Suga, H., Eiraku, M., Kadoshima, T., Yonemura, S., Takata, N., Oiso, Y., Tsuji, T., & Sasai, Y. (2016). Functional anterior pituitary generated in self-organizing culture of human embryonic stem cells. Nature Communications, 7(1), 10351–10351. https://doi.org/10.1038/ncomms10351
-
Qian, X., Nguyen, H. N., Song, M. M., Hadiono, C., Ogden, S. C., Hammack, C., Yao, B., Hamersky, G. R., Jacob, F., Zhong, C., Yoon, K., Jeang, W., Lin, L., Li, Y., Thakor, J., Berg, D. A., Zhang, C., Kang, E., Chickering, M., … Ming, G. (2016). Brain-Region-Specific Organoids Using Mini-bioreactors for Modeling ZIKV Exposure. Cell, 165(5), 1238–1254. https://doi.org/10.1016/j.cell.2016.04.032
-
Sakaguchi, H., Kadoshima, T., Soen, M., Narii, N., Ishida, Y., Ohgushi, M., Takahashi, J., Eiraku, M., & Sasai, Y. (2015). Generation of functional hippocampal neurons from self-organizing human embryonic stem cell-derived dorsomedial telencephalic tissue. Nature Communications, 6(1), 8896–8896. https://doi.org/10.1038/ncomms9896
-
Sakaguchi, H., Ozaki, Y., Ashida, T., Matsubara, T., Oishi, N., Kihara, S., & Takahashi, J. (2019). Self-Organized Synchronous Calcium Transients in a Cultured Human Neural Network Derived from Cerebral Organoids. Stem Cell Reports, 13(3), 458–473. https://doi.org/10.1016/j.stemcr.2019.05.029
-
Trujillo, C. A., Gao, R., Negraes, P. D., Gu, J., Buchanan, J., Preissl, S., Wang, A., Wu, W., Haddad, G. G., Chaim, I. A., Domissy, A., Vandenberghe, M., Devor, A., Yeo, G. W., Voytek, B., & Muotri, A. R. (2019). Complex Oscillatory Waves Emerging from Cortical Organoids Model Early Human Brain Network Development. Cell Stem Cell, 25(4), 558–569.e7. https://doi.org/10.1016/j.stem.2019.08.002